< back to main page
Abstract
About 60% of the heat transfer from the inner rooms of a building to the outside is due to radiation. Standard building materials have emissivity values around 0.9 which means they will emitt about 90% of their stored heat, and when exposed to heat radiation they will absorb about 90% of it. New wall paints have been developed with emissivity values ranging from 0.5 to 0.65. With these new paints heat losses in winter and heat gains in summer will be reduced as much as 30% depending on the degree of standard insulation and climatic region.
Short view on the history of paints
Like other building materials, have paints made a change in their specific properties and applications throughout the years. When we think of the first generation of paints, the early cave paintings, they had a more decorative and informative character. The second generation of paints had besides these characteristics another feature, the protection of the painted surface.
Just recently a third generation of paints has been introduced to the market, a low emissive (low-e) coating which not only controls the absorption of light in the visible region of the electromagnetic spectrum, it controls the absorption and reflection of heat radiation especially in the region of ambient temperatures. We know that white light can be divided into different colors with the aid of a prism. This is due to the fact that the different colors from blue to violet represent electromagnetic waves at different wavelengths.
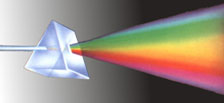
Spectral colors of light
Without special electronic equipment we only can see in this small part of the electromagnetic spectrum and so we only were interested in the visible properties of a paint besides the protective properties. The third generation of paints - the low-e coating - however has not only visual properties, it controls the reflectance and the emission of electromagnetic waves of the so covered surfaces in the unvisible, the thermal infrared region of the electromagnetic spectrum where objects on ambient temperature have their maximum radiation.
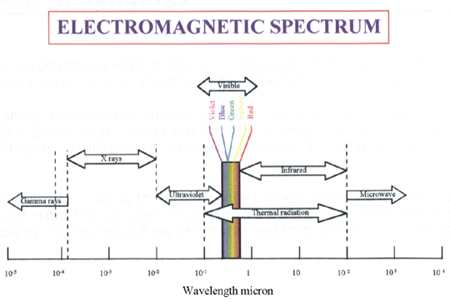
The electromagnetic spectrum
A brief introduction into the physics of thermal radiation
The expression thermal radiation shall be introduced with the help of some examples everybody knows from daily life.
We are not aware of thermal radiation unless it manifests itself visually such as radiation from the sun, a fire, or red-hot to white-hot metal. Even tiled stoves are associated with thermal radiation and in particular with comfortability although, when compared with the other events, there is no visible component in the thermal radiation of a tiled stove.
Fire can be taken as an example to more profoundly examine the radiation characteristics of such type of heat supply. Everybody is familiar with the effect that, on cooler evenings, only that part of the body is getting warm which is turned to the fire. If one sits there facing the fire, the back may remain unpleasantly cool, even though the front part of the body is already too hot. This means that air close to the fire is not heated up by the latter.
What is not illuminated by fire is also not heated up. That thermal radiation can be bundled up like the light of a torch by means of a reflecting mirror is also known from radiators which, to improve efficiency, when they are equiped with a reflector.
We are only aware of thermal radiation when the source of heat is clearly hotter than the environment. A reason for this can be found in the fact that we ourselves are a source of heat at a quite high temperature level. If a skin temperature of 34°C is assumed the radiated heat will result in 503 W/m². If, however, there is a temperature of 20°C in our environment, heat emission will be only 418 W/m². Consequently, 85 W/m² are taken away from us.
For better understanding, the difference between heat transport by air and heat transport by radiation is to be briefly explained. It was shown that thermal radiation spreads like light. Max Planck found that all bodies having temperatures above absolute zero (- 273° C) emit energy. As the temperature of the body increases, energy is emitted at higher frequency or shorter wavelength. The following graph shows the spectral emission of „blackbodies“ at increasing temperatures. A „blackbody“ is an ideal body which emits all of it’s energy so the emissivity is defined as 1.0.
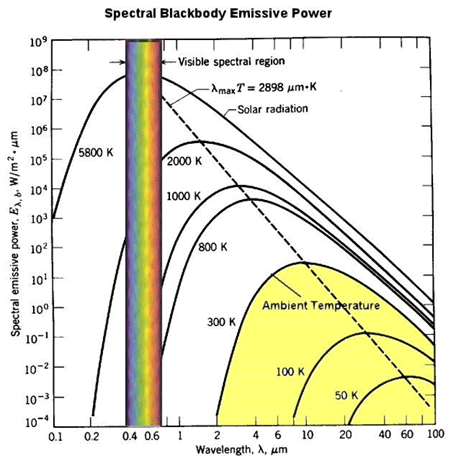
Spectral emissive power of blackbodies at different temperatures
Thermal radiant heat transfer is usually associated with the rate at which energy is emitted by matter because of its finite temperature. All matter above absolute zero (0 Kelvin = -273°C ) emits radiant energy in form of electromagnetic waves. In a building, the walls, furniture, floor, and ceiling all emit radiant energy. Outdoors, the sun, atmosphere, ground .... everything emits radiant energy in relation to their temperature. But especially when we think of the sun, they do not emit at the same wavelengths. Max Planck has determined the spectral distribution of a blackbody emitter. The spectral emissive power for a diffuse blackbody emitter is described by:

One knows the resulting Planck curves for blackbodies at different temperatures where the sun with a surface temperature of 5800 K has it’s maximum radiation at wavelengths from 0.4 to 0.6 µm in the visible region of the electromagnetic spectrum and blackbodies at ambient temperatures of 300 K (27° C) are radiating in the thermal infrared at wavelengths from 5 to 50 µm.
Another physical law concerning thermal radiant energy emission is the Stefan-Boltzmann law. It states that the heat flux emitted by an ideal radiator (i.e. a blackbody) is proportional to the the absolute temperature (in degrees Kelvin) to the fourth power. The proportionality constant σ is called the Stefan-Boltzmann constant.

Actual surfaces in the real world emit less radiant energy than the ideal blackbody. The ratio of the actual emissive power E to the emissive power of a blackbody Eb , at the same temperature is called the emissivity. It is defined by:

where ε is dependent on wavelength λ, angle δ and temperature T
The formula for the actual emissive power E = ε · σ · T4shows that the emissivity of a surface has a great impact on the emissive power of a surface. A wall with a surface temperature of 20° C and an emissivity of 0.95 will emit 397 W/m², and with an emissivity of 0.55 it is only 230 W/m². When the transmittance is zero the heat reflectance of a surface is described by

Spectral properties of common building materials
Most common building materials have emissivity values ranging from 0.85 to 0.95. The only exceptions are low-e glass windows. Here the lower surface emissivity of the inner sides of the glass panes reduce the radiative heat transfer between the inner and outer pane. A room with low emissive inner surfaces has much in common with the low-e windows as far as the radiative heat exchange is concerned. The convective heat exchange is different though. It will be discussed in this paper what effects occur when the emissivity of indoor walls and ceilings are modified to lower emissivity values.
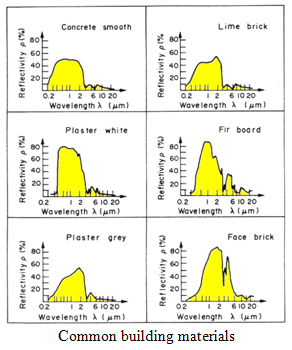
The above graphic shows the spectral reflectivity of different typical building materials. At the wavelengths of interest i.e. over 3 µm the reflectivity is very low and so the emissivity and absorbtion is very high, mostly over 90%. Which means these materials will absorb over 90% of the indoor generated heat radiation and lead it via heat conduction to the outside. For the summer climate when the sun heats up the outer walls, this means they will emit over 90% into the room.
Standard wall paints have the same characteristics as far as the thermal infrared region of interest is concerned. The next diagram shows the reflectivity in the thermal infrared of a standard wall paint compared to three different low-e wall paints.
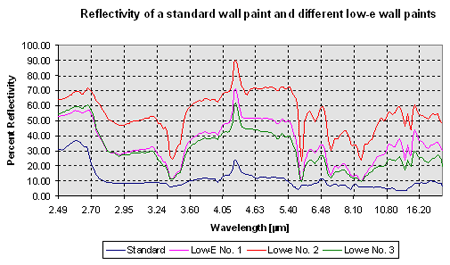
Reflectivity of a standard wall paint compared to different low-e wall paints
Effects of low emissive wall paints in winter and summer
As mentioned before, we only can see with our eyes in a very small portion of the electromagnetic spectrum. For other wavelengths than the visible we have to use special sensors to get an impression or to measure at these spectral regions. The following picture of a cup filled with hot coffie in front of a wall was taken with an infrared camera. The left side of the wall was painted with a low-e wall paint and the right side with a standrad paint.
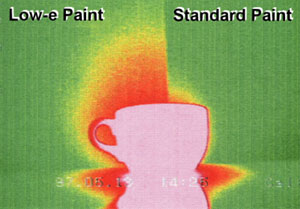
Thermal image of a hot cup of coffie in front of a low-e and standard wall
The infrared image shows that the heat radiation of the hot coffie cup is reflected by the side of the wall which is painted with a low-e paint. As mentioned hereinbefore, thermal radiation spreads like light. One has to imagine a lightbulb in the middle of a room, whose walls are painted black. There is a desk to work on in one corner of the room. As light is absorbed by the black walls, there is not enough light at this place of the room. However, if the walls are white i.e. painted in a reflective manner, the room's brightness will be increased by multiple reflection from the walls.
Standard wall paints have the same effect for thermal radiation as the black paint has for light. over 90% of thermal radiation is absorbed by the walls and conducted in the wall to the outside. Low-e wall paints will reflect part of the heat generated in the room back into it.
Now, what happens in summer, when the walls of a house are painted with a low-e wall paint?
During a longer period with high temperatures, the walls will heat up more slowly or rapidly, depending on their heat storage capacity and the walls will start radiating heat into the rooms. In the evening or at night, while it is already pleasantly cool outside, it is still unbearably hot in some parts of the house even though there was an exchange of air with cooler air from outside or from the air condition.
However, the exchange of air had not much influence on the thermal radiation of the walls. At a temperature of the wall of 30° C for example, the resultant thermal radiation is 478 W/m². This heat cannot be simply vented away. It can be reduced only by increasing the cooling power of the air condition. In other words, the air condition has to compensate the heat radiation of the walls, so the air must be cooled down to an unpleasant degree. This is the only way persons in the room can release their metabolic heat.
If the inside walls are painted with low-e wall paint, there will be a much more positive situation. In accordance with Max Planck’s law concerning the thermal radiation, the heat emitted from the walls can be reduced when compared with normally painted walls. The infrared image below shows a hot steel panel painted with a low-e paint on the left side. The color code of the camera, blue for cold and red to yellow for hot, shows that the standard painted side radiates much more heat.
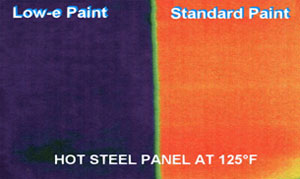
Thermal image of a hot steel plate
Heat transfer coefficient and thermal comfort
There are two ways an indoor low emissive wall paint will have an effect on the energy consumption in a building. First it will raise the thermal resistance of the outer walls of the building in winter and summer, and second it will increase the thermal comfort in a building in winter and summer by changing the mean radiant temperature. The thermal resistance value Rth for an outside wall is given by:

A lower emissivity of the wall will result in a smaller radiation heat transfer coefficient hrad,i and a higher thermal resistance Rth,tot of the wall. When all the walls and the ceiling of a room have a lower emissivity than normal it is not only the smaller radiation heat transfer coefficient hrad,i which reduces the heat losses or gains through the wall it is the radiative heat flow from the warmer inner walls to the colder outer wall which will be reduced as well. In Summer it has the same effect only the direction of the heatflow is changed.
The rate of heat transport between two surfaces that see nothing else or that are configured that one encloses the other is given by:
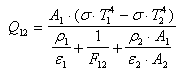
Normal walls with an emissivity around 0.9 to 0.95 will only have an reflection of 5 to 10% which can be neglected. With low emissive walls heat reflection can increase up to 50% . In this case multiple reflection will occur which has to be taken into account when the radiative exchange is calculated. Normally this is done with an iterative working computer model.
Depending on the emissivity of the low-e wall coating the total heat transfer coefficient for the inner side of an exterior wall htot,i = hrad,i + hcon,i can be lowered by 31.5% for an emissivity of 0.62 and 36.8% for an emissivity of 0.53 compared to the htot,i for a standard wall paint. For an example calculated by the Fraunhofer-Institute for Building Physics, Stuttgart Germany (1) the R-value of a brickwall could be increased by 8% and for the emissivity of 0.53 to about 11%.
The second way low emissive wall coatings will have an effect on buildings energy consumption is an increase in thermal comfort. The formulas of the ASHRAE comfort recommendations where the thermal balance of a person in relation to an enclosure is described include besides many other parameters a mean radiant temperature Tr for the enclosure. The mean radiant temperature Tr has an effect on the so-called operative temperature Top which describes whether a person feels to cold or to hot in a room.
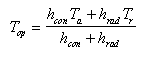
When the walls of an enclosure have a lower emissivity than normal, the air temperature in the enclosure can be decreased in winter and increased in summer with the same level of thermal comfort. The german IWU Institut Wohnen und Umwelt (Institute for Residential Housing and Environment) has calculated the increase of the operative temperature in winter due to low emissive walls with 0.7° C. (2) They have stated that the air temperature in the house can be lowered by this value with still a better thermal comfort.
This has a significant effect on energy savings as well. Not only the heat transmission losses or gains through walls, windows and roof are decreased, the heat losses in winter or heat gains in summer due to air exchanges are reduced as the difference between indoor and outdoor air temperatures becomes smaller.
Further calculations and measurements show that in a house where walls and ceilings have emissivity values lower than normal, the operative temperature Top will change remarkably due to the position of a person in a room and depending on the size of a room. The smaller a room or the closer a persons position to the low-e wall, the more of the persons metabolic heat is reflected back and so effects the operative temperature in a positive way. The lower the emissivity value the higher the effects will be.
This looks like an advantage in winter, and it seems to look like a disadvantage in summer when the heat comes through the exterior walls into the room. But a simple energy balance calculation shows that the low-e wall has a positive effect in summer too.
Energy savings due to low-e interior wall paints
Depending on the location of a house, which determines the climatic conditions and the costs for gas and electricity, and the grade of insulation, energy savings due to low-e interior wall coatings will range from 15 to 30%. A normally insulated 1970 Rambler at Philadelphia, PA will have for example about 15% savings (see next diagram) and a 119 m2 non insulated concrete building at Chikago IL will have about 30% of energy savings.
The diagram shows the heating and cooling costs by of a 1970 Rambler at Philadelphia.
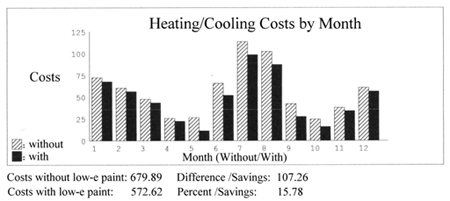
Localized energy savings calculation
Besides the calculations the energy savings have already been proved by users of low-e interior wall paints by comparing the gas and electricity bills before and after the application of the low-e paint. Comparing the testimonials with the calculations based on the existing computer models, the calculations are on the conservative side. Especially the testimonials concerning the increase of thermal comfort in a house have been far above what would have been expected from the standard thermal comfort calculations.
But as personal testimonials are only considered to be objective when a statiscal big group of persons come to the same results, the described thermal comfort test, where at least the temperature can be measured which a person would feel, is important to back the calculations.
It is possible now to calculate from the difference of the operative temperature in a low-e painted room and the standard painted room the energy savings for the whole house. Furthermore it is planned for further tests to control the air conditioning of a house with the measured operative temperature of the simulated bodies.
Exterior low-e wall paints
At the Fraunhofer-Institute for Buildings Physics, Holzkirchen, Germany, exterior low-e wall paints are in a field test since summer 97. These tests are financed with 50% by the Government of Bavaria and 50% by interested industry. As the last winter has been very mild in Germany and so is not representative for normal german winters, the tests will go on at least another winter period.
Low-e paints at two different colors are tested in comparison to standard paints on a brick wall and on a brick wall with rockwool insulation. During the time period from December 97 until March 98 the savings of heat transmission losses due to the exterior low-e paint have been measured with 14%.
The photograph below shows the test building at the Fraunhofer-Institute for Buildings Physics at Holzkirchen, Germany. Only the light red and white panels on the right side of the building belong to the test. Right in front of the wall one can see the measurement setup for solar irradiance and thermal infrared background.
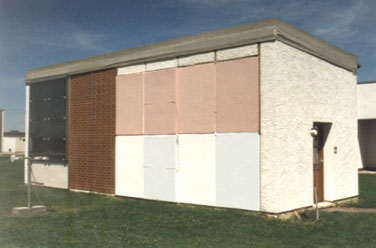
Test building at the Fraunhofer-Institute for Building Physics Holzkirchen
The diagram below shows some results of heat flow measurements through four different panels during a week with mostly clear weather.
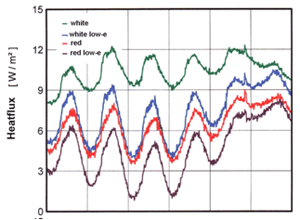
Heatflow through 4 panels of the test wall for the period of one week
Due to the solar irradiance the heat losses are always at minimum short after noon. At night the heat losses are at maximum. The last two days of this week the days and nights have been mostly cloudy. The differences in heat flux due to the low-e paint are smaller here. This means that the exterior low-e paints have a greater effect when the sky is clear. During the daytime they catch more solar energy without loosing all of it by emission and during the night, when the clear sky is much colder than a cloudy sky they do not emit as much as a standard paint.
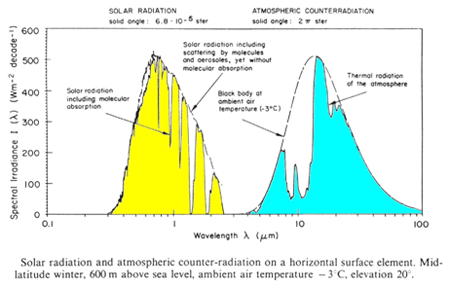
Spectral irradiance of the sun and the atmosphere
The above diagram shows the spectral irradiance of the sun and the clear sky. That the amount of energy looks almost the same is due to the fact that the sun radiates only at an angle of 6.8 · 10-6 ster and the sky with 2 π ster. The diagram shows further that the sun radiates most of it’s energy in the visible region of the electromagnetic spectrum but there is still about 50% of energy in the unvisible near infrared. The diagram of the clear sky shows that there is only very low radiation from 8 to about 12 µm. Here the atmosphere is very open to the cold space. Over 100 W/m² are withdrawn from earth under clear sky conditions.
An energy efficient exterior wall paint should be highly absorbing in the solar region and it should have a low emissivity in the thermal infrared especially from 8 to 13 µm where the atmosphere is open. Black paints are highly absorbing in the visible and even the near infrared, but nobody would like to live in a black painted house. So the paint must be light in color for the visible region but absorbing at the near infrared region over 0.7 µm where the sun still radiates about 50% of energy.
New developments in the field of intelligent paints show that it is possible to absorb solar energy with an almost white paint. Furthermore these paints are low emissive in the thermal infrared region so they do not loose all of the collected energy by emission.
The following diagram shows the spectral reflectance of an exterior white low-e paint compared to a standard white paint. One can see the difference that even with a degree of whiteness which is not to bad (high reflectance from 0.4 to 0.7 µm) this paint absorbs much more in the near infrared than the standard white paint. In the thermal infrared at 8 to over 13µm it is low emissive which is shown as higher reflectance than the standard paint. Further developments will be neccassary to improve the spectral properties of the exterior low-e paint.
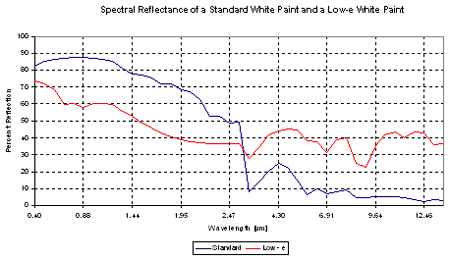
Spectral reflectance of a low-e and a standard exterior wall paint
Unlike the interior low-e paints were it is obvious that they increase thermal comfort and decrease energy bills in cold and hot climates, the behavior of an exterior low-e paint for vertical walls under hot climate has not finally been investigated.
Outlook
It could be shown that low-e interior wall paints have a significant effect on energy savings and thermal comfort in a house. Depending on the location and the degree of insulation of a house, savings of over 15% can be achieved just by applying a different paint with new features on the walls. Interior low-e walls paints can be applied in all climatic regions. They improve the room climate in winter and in summer.
Exterior low-e wall paints are still under test. As first measurement results show, another 15% of energy savings can be achieved. The field tests in Germany will at least cover the next winter period 98/99. It still has to be investigated for which climatic regions exterior low-e wall paints can be recommended.
< back to main page
For further product information on low emissive wall coatings,
please contact IPS (Mr. Gerd Hugo) directly:
e-mail: info [at] ips-innovations.com
|